Several definitions exist for the term periglacial. The earliest definitions suggested that these geomorphic environments were located at periphery of past Pleistocene glaciers. In these environments, the landscape is dominantly influenced by frost action. However, frost action also influences landscapes that were not at the margin of ancient glaciers. For this reason, we should use a broader definition of this term. This definition suggests that in a periglacial environment the effects of freezing and thawing drastically modify the ground surface. Types of modification include the displacement of soil materials, migration of groundwater, and the formation of unique landforms. More than a third of the Earth's terrestrial surface can be included in this definition.
Permafrost
Permafrost is a condition where a layer of soil, sediment, or rock below the ground surface remains frozen for a period greater than a year. Permafrost is not a necessary condition for creating periglacial landforms. However, many periglacial regions are underlain by permafrost and it influences geomorphic processes acting in this region of the world.
Permafrost is found in about 25 percent of the Earth's non-glaciated land surface (Figures 10ag-1 and 10ag-2). It occurs wherever the mean annual ground temperature is less than 0° Celsius. The southern limit in Canada generally correlates with an annual surface air temperature of -8° Celsius. In some areas, permafrost can be up to 1500 meters deep. Common depths of permafrost are several hundred meters. Most deposits of permafrost have an upper active layer that is between 1 to 3 meters thick. This active layer is subject to a cyclic thaw during the summer season.
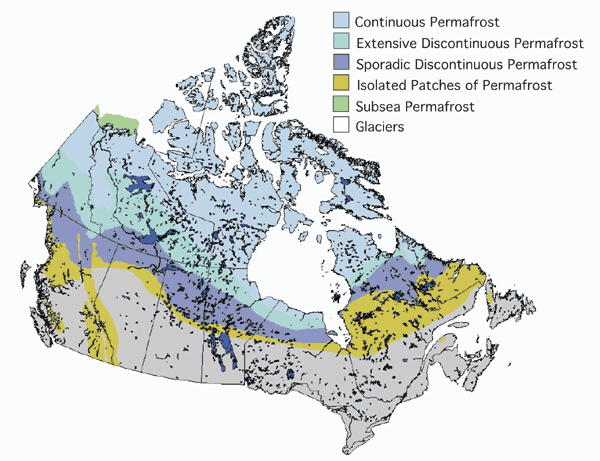
Figure 10ag-1: Distribution of the various types of permafrost in Canada. More than 50% of Canada is covered by some form of permafrost. This map does not identify areas of alpine permafrost (Source:Natural Resources Canada - Terrain Sciences Division - National Permafrost Database).
In some places, localized unfrozen layers or taliks are located on top, underneath, or within masses of permafrost (Figure 10ag-2). Often in continuous permafrost areas, taliks are found under lakes because of the ability of water to store and vertically transfer heat energy. The vertical extent of the taliks found under lakes is related to the depth and volume of the overlying water body. Larger water bodies can store and transfer more heat energy downward.
A closed talik is unfrozen ground that is found within a mass of permafrost. Closed taliks can develop when lakes fill in with sediment and become bogs. With the removal of the open water, summer solar radiation is now being received by a surface with a lower specific heat and poor vertical heat transfer. As a result, soil near the surface begins to freeze solid encasing a zone of unfrozen soil in permafrost. Closed taliks can also form because of groundwater flow.
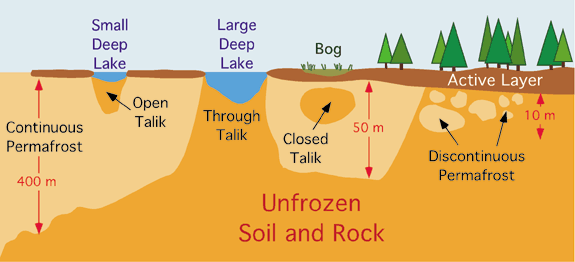
Figure 10ag-2: Vertical cross section of the transition zone between continuous and discontinuous permafrost. The graphic also shows the various types of talik or unfrozen ground. Open talik is an area of unfrozen ground that is open to the ground surface but otherwise enclosed in permafrost. Through talik is unfrozen ground that is exposed to the ground surface and to a larger mass of unfrozen ground beneath it. Unfrozen ground encased in permafrost is known as a closed talik.
Five different types of permafrost have been recognized (Figures 10ag-1 and 10ag-2). Continuous permafrost exists over the landscape as an uninterrupted layer. Zones of permafrost with numerous scattered small thawed areas are called discontinuous permafrost. Discontinuous permafrost in usually exists as an extensive marginal zone at the edge of continuous permafrost. Sporadic permafrost occurs where small islands of permafrost are scattered in generally unfrozen areas. Alpine permafrost is found at higher elevations in areas outside where continuous or discontinuous permafrost is common. Sub sea permafrost is frozen ground that exist in the sediments beneath seawater. Large areas of sub sea permafrost exist along the northern coastal edge of Russia, Alaska, and parts of northern Canada.
Periglacial Processes: Weathering
Locations that have a periglacial environment are characterized by the presence of large quantities of angular, fractured rock (Figure 10ag-3). The angular nature of these deposits suggests that the process responsible for the rock fracturing is the crystallization of water. The quantity of the deposits indicates that the frost weathering process operates over and over again in repeated cycles of freeze-thaw. In fact, repeated thawing allows further fracturing because the liquid water is able to fill newly developed cracks.
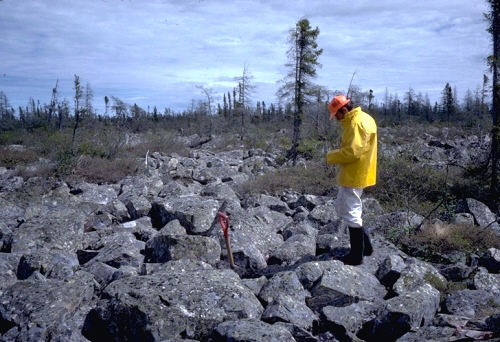
Figure 10ag-3: Frost-shattered granite bedrock (felsenmeer), northern Manitoba. This is a close-up of frost-shattered bedrock, consisting of angular blocks of Precambrian granite. Extensive areas of blocks are called felsenmeer. The shattering has occurred in permafrost terrain, near treeline. (Source: Natural Resources Canada - Terrain Sciences Division - Canadian Landscapes).
The critical temperature for the development of frost induced fracturing is between -4 and -15° Celsius. Scientists have also discovered that relative slow rates of cooling produce the greatest quantity of fracturing stress. Measurements for marble and granite indicate that a rate of cooling between -0.1 and -0.5° per hour is optimal.
Periglacial Processes: Ground Ice
Surface soils and sediments in periglacial environment are frequently influenced by a variety of different types of ground ice. Some of these masses of ground ice can be as much as 30 meters across. The most common form of ground ice is pore ice. Pore ice develops in the pore spaces between soil and sediment particles where liquid water can accumulate and freeze. Another common form of ground ice is needle ice. Needle ice consists of groups of narrow ice slivers that are up to several centimeters long. They normally form in moist soils when temperatures drop below freezing overnight. Needle ice plays an active role in loosening soil for erosion and tends to move small rocks upward to the soil surface. On sloped surfaces, needle ice can also enhance soil creep by moving soil particles at right angles to the grade.
Ice wedges are downward narrowing masses of ice that are between 2 to 3 meters wide at the base and extend below the ground surface up to 10 meters. It is believed that they form when a seasonal crack in the ground forms in the winter. During this season, extreme cold temperatures can cause soil contraction. Soil and ice, like other solid materials, contracts as temperatures drop way below 0° Celsius. At first, the crack is several millimeters wide and about a meter deep. When temperatures warm up in the summer, liquid water from the active layer fills the crack. This water then refreezes because the fracture extends into the sub-zero permafrost. The freezing of the water results in a volumetric expansion of about 9%.
This expansion then increases the width and depth of the fracture. At the same time, the soil layers adjacent to the developing ice wedge are pushed outward and upward creating a slight hill at the surface. The processes just described cause the ice wedge to increase in size with each passing year. Each winter new cracks form in the ice wedge because of contraction. In the summer, more liquid water seeps in to the cracks to become frozen and the summer warming results in the surface mound getting bigger (Figure 10ag-4). Each cycle of ice addition can be identified as narrow layers called foliations. In arid cold environments, some ice wedges can accumulate quantities of wind blown sand within their winter cracks. These particular types of ground ice are often given the special name sand wedges.
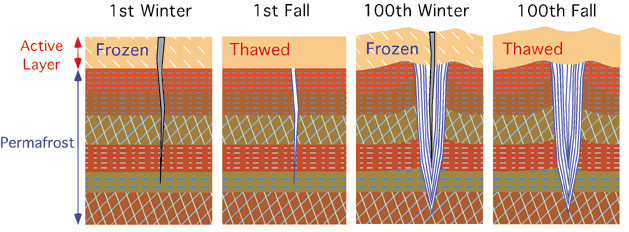
Figure 10ag-4: The following series of graphics showing the evolution of ice wedges. The development of an ice wedge begins with cold temperatures in winter forming a crack in the ground surface that is about a meter deep and a few millimeters wide. In the warmer months, melting of the active layer liberates liquid water which flows into the crack. This water then freezes on contact with the permafrost. The cycle just described repeats itself year after year with new cracks forming in the developing ice wedge. The two graphics on the right show a well developed ice wedge after many cycles of development.
Segregated ice are masses of almost pure ice that grow within permafrost because of liquid water diffusion. Many forms of segregated ice exist as extensive horizontal layers. In this form, they are sometimes called ice lenses. In the development of these features, water migrates under some type of pressure from unfrozen parts of the soil to the ice mass. When the liquid water comes in contact with the segregated ice mass it freezes enlarging the feature. Water diffusion can be caused by temperature and pressure gradients, gravity, and by the movement of groundwater under pressure. Most masses of segregated ice are found just below the active layer. The pressure exerted by overlying sediments controls the growth of segregated ice.
Periglacial Processes: Mass Movement
During the warmer seasons, mass movement can be a common phenomenon in periglacial environments. It usually occurs in four forms: solifluction; gelifluction; frost creep and rockfalls. Solifluction is the slow downslope flow of soil and sediment that is saturated with water. This process can occur on very shallow grades. The common sign of this form of mass movement is the presence solifluction lobes, tongue like semi-mixed surface deposits. In periglacial environments, solifluction is confined to times when temperatures are well above zero and free liquid water is available in the active layer. Solifluction is very common when surface sediments are poorly drained and quite saturated with water. Gelifluction is a form of solifluction where the moving materials slide over a slick permafrost layer.
Frost creep is the slow down slope movement of soil and sediment because of frost heaving and thawing (Figure 10ag-5). The process begins with the freezing of the ground surface elevating particles at right angles to the slope. The particles are elevated because cold temperatures causes water in between particles to freeze and expand. In the warm season, thawing causes the ice to convert back to liquid water and the contracting surface drops the particles in elevation. This drop, however, is influenced by gravity causing the particle to move slightly downslope.
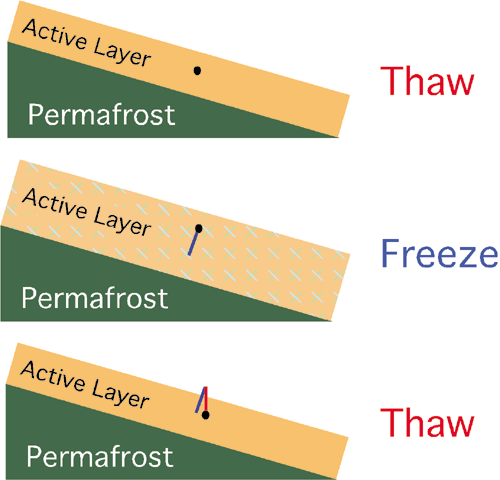
Figure 10ag-5: The following series of graphics show how frost creep moves soil particles gradually downslope. The top graphic shows the position of a single particle in a thawed active layer above a zone of permafrost. When cold temperatures occur the active layer freezes. This freezing causes the water between the soil particles to expand making the layer thicker. The expansion processes also moves the illustrated soil particle up. This movement occurs perpendicular to the slope. With the return of warmer temperatures, the active layer contracts as the ice between soil particles melts. However, this contraction is controlled by the force of gravity causing the illustrated soil particle to move forward as it drops.
Exposed rock outcrops are common in periglacial regions of the world. Cold temperatures experienced here do not favor the development of an extensive layer of soil and regolith over bedrock. As a result, exposed rock on slopes is subject to this region's harsh environment. Extreme variations in temperature can cause the fracturing of rock along natural bedding planes and joints leading to rockfalls. This fracturing is mainly due to frost wedging and insolation weathering
Periglacial Processes: Erosion
Processes of erosion and deposition in periglacial parts of the world tend to have their own unique character. These characteristics are related to the importance of freeze-thaw action, the presence of strong winds, and the fact that the warm season is very short. As a result, three forms of processes tend to dominate in periglacial environments: nivation; eolian erosion and deposition; and fluvial erosion and deposition.
Nivation is the localized form of erosion associated with isolated patches of snow that remain through the summer season. It involves three separate processes: frost weathering at the margins of snow patches; meltwater erosion; and gelifluction. Snow patches that persist through the warm season create an environment for physical weathering at their margins. Temperatures at the margins of the snow fluctuate between above and below 0° Celsius diurnally. As a result, water in the cracks of rocks located by the snow changes from liquid to solid many times, quickly creating a mass of small fragments. If the snow patch is on a grade, meltwater along the base of the snow patch will transport the weathered rock fragments downslope. The snow patches can also insolate the soil and sediments that lay underneath it. The effects of insolation can raise the temperature of these materials to above freezing when combined with seeping water from melted snow. Once thawed and saturated, the sediments may begin moving by gelifluction if a slope exists.
A common feature created by nivation is a nivation hollow. These features have been known to develop under snow patches in just a few seasons. The usual location of these features in the Northern Hemisphere is on a slope that faces northeast. This orientation is of course most protected from the warming effects of Sun's rays. The development of the hollows requires two ingredients: a snow patch that returns to the same area year after year and a slope to allow for erosional transport of material out of the developing depression. The process begins with a patch of snow.
Around the edges of this patch, physical weathering and frost heaving begins to separate particles for erosion. Running water then picks up the loose particles and carries them off. Material is also removed from the developing hollow by gelifluction. As the summer season progresses, the patch of snow reduces in size and the excavation of material continues inward. Enlargement of the hollow involves several different mechanisms. Sometime in the following year, the boundary of the snow mound and depression will once again be inline and frost weathering will eat away at the hollow's edge. The edge of the hollow is also preferentially eroded because the micro-slope creates localized instabilities and focuses the entrainment potential of flowing water.
Strong winds are a characteristic of periglacial areas. These winds often move large quantities of loose sediment and soil. This is especially true during the summer months when increases in stream discharge, drying of sediments, and the melting of snow and ice make more material available for eolian transport. Some of the depositional features that are created include sand dunes, loess accumulations, and sand sheets.
The discharge of most streams in periglacial regions exhibits particular temporal patterns. Most of the flow tends to occur during a period of weeks when snowmelt occurs. The discharge patterns of these streams may also show wide fluctuations that are timed diurnally. This is unlike temperate and tropical streams where discharge is taking place throughout the year. The concentration of discharge in a short period leaves some peculiar features. These short-lived streams tend to have poorly developed shallow braided channels. When discharge is suddenly reduced, large quantities of gravel and boulders are left on the landscape where the flow was taking place. The final unique characteristic of periglacial streams is that their channels can be beaded with deep pools. Beaded channels develop when a stream passes over a network of ice wedges. Thermal properties of the flowing water cause the ice wedges to melt producing pools.
Periglacial Landforms
The surface of periglacial areas is often characterized by the presence of ground materials arranged in a variety of symmetrical, geometric shapes (Figures 10ag-6 and 10ag-7). These features are collectively known as patterned ground. Shapes of patterned ground can include stripes, steps, circles, polygons, and nets. Sometimes one pattern can morph into another shape. Researchers have discovered that a single process cannot explain the various forms observed. Many of these features appear to be caused by freeze-thaw action selectively moving coarse particles to the edge of the shape or to its surface. Some polygon forms seem to be caused by the same thermal processes that create ice wedges. Yet, other types of pattern ground still remain not fully explained.
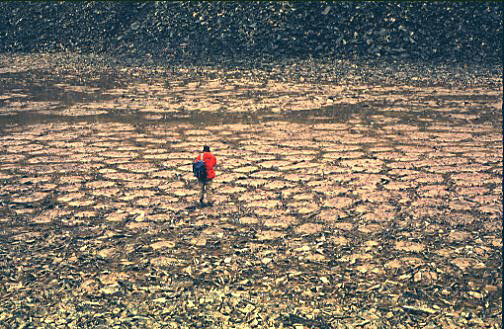
Figure 10ag-6: Stone circles on Melville Island, Northwest Territories, Canada. The patterns on the ground consist of circular arrangements of slabs (foreground) and small domes of mud with stony rims. The features were produced by the churning action of frost forming in unsorted glacial till and regolith. Countless freeze-thaw cycles sorted the surface debris, continually heaving the finer matter to the surface, and leaving the coarser fragments around the edges. (Source: Natural Resources Canada - Terrain Sciences Division - Canadian Landscapes).
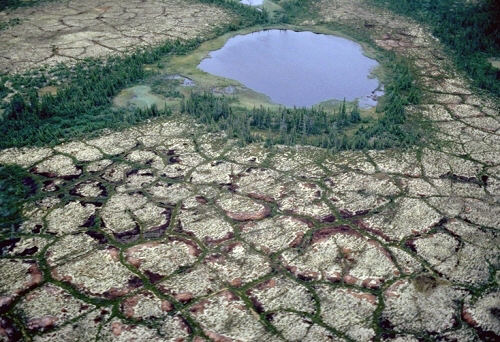
Figure 10ag-7: Ice-wedge polygons in peatland, Hudson Bay Lowlands, Manitoba. Splendid examples of ice-wedge polygons, a form of patterned ground, are shown above. They occur in the permafrost peatlands of the Hudson Bay Lowlands, which are composed mainly of dry sphagnum. Brown polygons mark the location of massive ice wedges that extend from the surface down to 2 or 3 m. (Source: Natural Resources Canada - Terrain Sciences Division - Canadian Landscapes).
Palsas are low permafrost mounds with cores of layered segregated ice and peat (Figure 10ag-8). They are normally 1 to 7 meters high, 10 to 30 meters wide, and 15 to 150 meters long. They are also most common at the southern margin of the discontinuous permafrost zone. Palsas are believed to form when areas of reduced snow cover allow frost to penetrate more deeply into an unfrozen peat bog. This frost freezes the water in the peat forming an initial ice layer. The layer then grows in size over time as water migrates under some type of pressure from unfrozen parts of the peat to the surface of the growing ice mass.
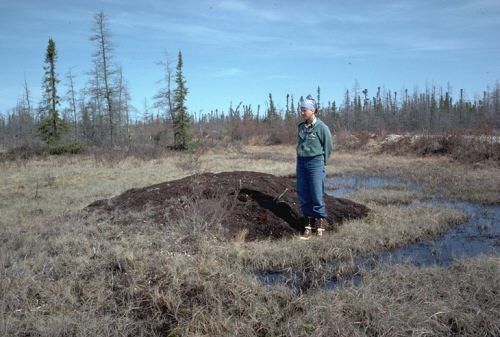
Figure 10ag-8: Emerging palsa in a fen bog near Churchill, Manitoba. This palsa is in the initial stages of development and is growing by absorbing some of the water in the surrounding wetland. As segregated ice builds up inside this feature, the water-loving vegetation growing on the peat is pushed up above the water table. This action causes the vegetation to die off, resulting in the bare brown peat surface on top of the mound. (Source: Natural Resources Canada - Terrain Sciences Division - Canadian Landscapes).
Pingos are ice-cored hills with a height between 3 to 70 meters and a diameter between 30 to 1000 meters (Figure 10ag-9). Most pingos are circular in shape. Smaller pingos tend to have a curved top. Large ones usually have exposed ice at their top and the melting of this ice often forms a crater. Sometimes the craters are filled with water forming a lake. The ice at the core of pingos is thought to accumulate because of cryostatic pressure and artesian groundwater flow.
The development of a cryostatic pingo begins with a lake with no permafrost beneath it (talik). The lake then gradually fills in with sediment and invading permafrost isolates the remaining water in the lake's sediments. Continued inward and downward freezing of the old lake sediments generates enough pressure to move pore water upward. This pore water then begins to freeze to form a segregated mass of ice at the core of the developing pingo. Artesian pingos develop when a supply of groundwater is channeled to a particular location where it freezes just below the ground surface.
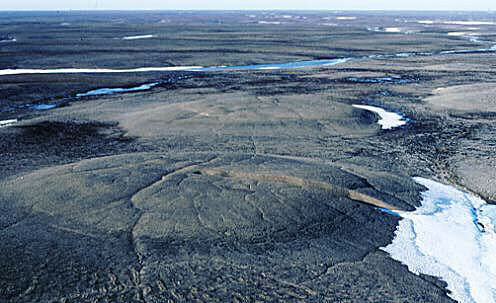
Figure 10ag-9: Pingos on Prince Patrick Island, Northwest Territories, Canada. These features are up to 100 meters across. Eventually the ice core will grow to such a size that it will rupture the sediment cover and become exposed. Scientists are unsure where all of the free water is coming from and how it is conducted through the frozen sediments and rock to make these features grow. (Source: Natural Resources Canada - Terrain Sciences Division - Canadian Landscapes).
Some pingos are still actively growing. The maximum rates of vertical growth of young pingos can be as high as 1.5 meters per year. The dating of pingos has reveled that these features are generally less than 10,000 years old. Many small ones in the Arctic have ages that are less than a few hundred years old. Scientists estimate that several thousand exist in the periglacial regions of the Northern Hemisphere.
CITATION
Pidwirny, M. (2006). "Periglacial Processes and Landforms". Fundamentals of Physical Geography, 2nd Edition. 10/1/2012. http://www.physicalgeography.net/fundamentals/10ag.html