Simple Model of Global Circulation
We can gain an understanding of how global circulation works by developing two simplified graphical models of processes that produce this system. The first model will be founded on the following simplifying assumptions:
- The Earth is not rotating in space.
- The Earth's surface is composed of similar materials.
- The global reception of solar insolation and loss of longwave radiation cause a temperature gradient of hotter air at the equator and colder air at the poles.
Based on these assumptions, air circulation on the Earth should approximate the patterns shown on Figure 7p-1. In this illustration, each hemisphere contains one three-dimensional circulation cell.
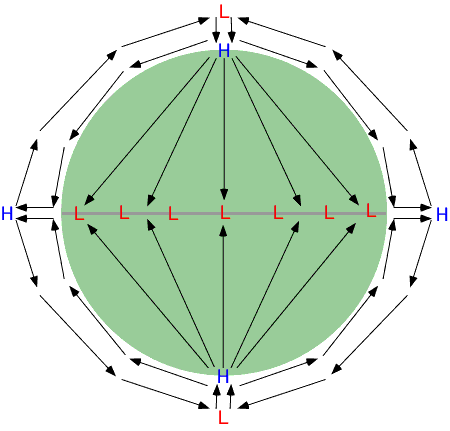
Figure 7p-1: Simplified one-cell global air circulation patterns.
As described in the diagram above, surface air flow is from the poles to the equator. When the air reaches the equator, it is lifted vertically by the processes of convection and convergence. When it reaches the top of the troposphere, it begins to flow once again horizontally. However, the direction of flow is now from the equator to the poles. At the poles, the air in the upper atmosphere then descends to the Earth's surface to complete the cycle of flow.
Three Cell Model of Global Circulation
If we eliminate the first assumption, the pattern of flow described in the model above would be altered, and the meso scale flow of the atmosphere would more closely approximate the actual global circulation on the Earth. Planetary rotation would cause the development of three circulation cells in each hemisphere rather than one (see Figure 7p-2). These three circulation cells are known as the: Hadley cell; Ferrel cell; and Polar cell.
In the new model, the equator still remains the warmest location on the Earth. This area of greater heat acts as a zone of thermal lows known as the intertropical convergence zone (ITCZ). The Intertropical Convergence Zone draws in surface air from the subtropics. When this subtropical air reaches the equator, it rises into the upper atmosphere because of convergence and convection. It attains a maximum vertical altitude of about 14 kilometers (top of the troposphere), and then begins flowing horizontally to the North and South Poles. Coriolis force causes the deflection of this moving air in the upper atmosphere, and by about 30° of latitude the air begins to flow zonally from west to east. This zonal flow is known as the subtropical jet stream. The zonal flow also causes the accumulation of air in the upper atmosphere as it is no longer flowing meridionally.
To compensate for this accumulation, some of the air in the upper atmosphere sinks back to the surface creating the subtropical high pressure zone. From this zone, the surface air travels in two directions. A portion of the air moves back toward the equator completing the circulation system known as the Hadley cell. This moving air is also deflected by the Coriolis effect to create the Northeast Trades (right deflection) and Southeast Trades (left deflection). The surface air moving towards the poles from the subtropical high zone is also deflected by Coriolis acceleration producing the Westerlies. Between the latitudes of 30 to 60° North and South, upper air winds blow generally towards the poles.
Once again, Coriolis force deflects this wind to cause it to flow west to east forming the polar jet stream at roughly 60° North and South. On the Earth's surface at 60° North and South latitude, the subtropical Westerlies collide with cold air traveling from the poles. This collision results in frontal uplift and the creation of the subpolar lows or mid-latitude cyclones. A small portion of this lifted air is sent back into the Ferrel cell after it reaches the top of the troposphere. Most of this lifted air is directed to the polar vortex where it moves downward to create the polar high.
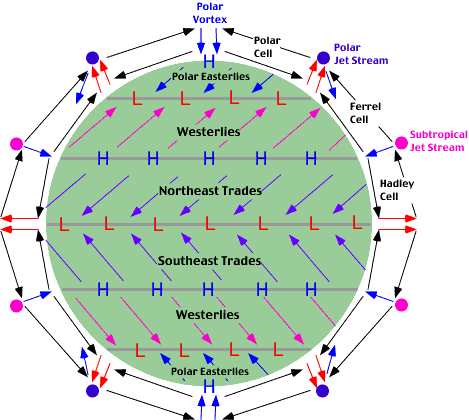
Figure 7p-2: Simplified global three-cell surface and upper air circulation patterns.
Actual Global Surface Circulation
Figure 7p-3 describes the actual surface circulation for the Earth as determined from 39 years of record. Average circulation patterns can be viewed on this animation for every month of the year. The circulation patterns seen differ somewhat from the three cell model in Figure 7p-2. These differences are caused primarily by two factors. First, the Earth's surface is not composed of uniform materials. The two surface materials that dominate are water and land. These two materials behave differently in terms of heating and cooling causing latitudinal pressure zones to be less uniform. The second factor influencing actual circulation patterns is elevation. Elevation tends to cause pressure centers to become intensified when altitude is increased. This is especially true of high pressure systems.
Figure 7p - 3 GLOBAL SURFACE CIRCULATION
Atmosphere pressure values are adjusted for elevation and are described relative to sea-level. The slider at the bottom of the image allows you change the time of month. Pressure values are indicated by color shading (see the legend in the graphic). Blue shades indicate pressure lower than the global average, while yellow to orange shades are higher than average measurements. Wind direction is shown with arrows. Wind speed is indicated by the length of these arrows (see the legend on the graphic).
Figures 7p-4 and 7p-5 are single frames from the animation above for January and July, respectively. On these modified graphics, we can better visualize the inter tropical convergence zone (ITCZ), subtropical high pressure zone, and the sub polar lows. The intertropical convergence zone is identified on the figures by a red line. The formation of this band of low pressure is the result of solar heating and the convergence of the trade winds. In January, the inter tropical convergence zone is found south of the equator (Figure 7p-4). During this time period, the Southern Hemisphere is tilted towards the Sun and receives higher inputs of shortwave radiation.
Note that the line representing the inter tropical convergence zone is not straight and parallel to the lines of latitude. Bends in the line occur because of the different heating characteristics of land and water. Over the continents of Africa, South America, and Australia, these bends are toward the South Pole. This phenomenon occurs because land heats up faster then ocean.
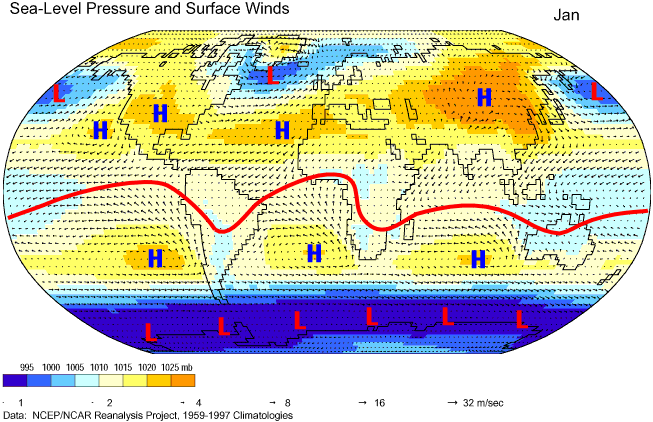
Figure 7p-4: Mean January prevailing surface winds and centers of atmospheric pressure, 1959-1997. The red line on this image represents the intertropical convergence zone (ITCZ). Centers of high and low pressure have also been labeled. (Source of Original Modified Image: Climate Lab Section of the Environmental Change Research Group, Department of Geography, University of Oregon - Global Climate Animations).
During July, the intertropical convergence zone (ITCZ) is generally found north of the equator (Figure 7p-5). This shift in position occurs because the altitude of the Sun is now higher in the Northern Hemisphere. The greatest spatial shift in the ITCZ, from January to July, occurs in the eastern half of the image. This shift is about 40° of latitude in some places. The more intense July Sun causes land areas of Northern Africa and Asia rapidly warm creating the Asiatic Low which becomes part of the ITCZ. In the winter months, the intertropical convergence zone is pushed south by the development of an intense high pressure system over central Asia (compare Figures 7p-4 and 7p-5). The extreme movement of the ITCZ in this part of the world also helps to intensify the development of a regional winds system called the Asian monsoon.
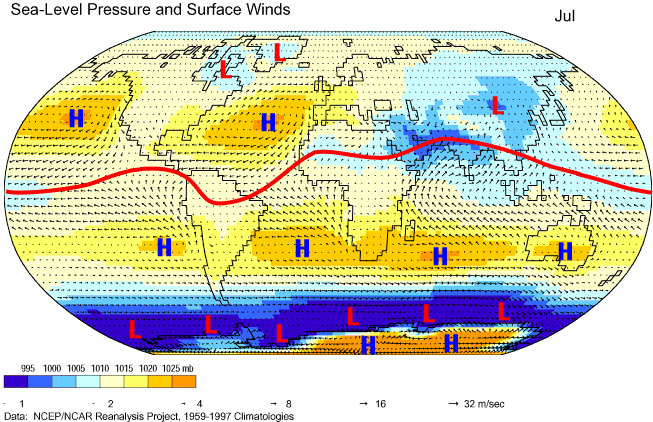
Figure 7p-5: Mean July prevailing surface winds and centers of atmospheric pressure, 1959-1997. The red line on this image represents the intertropical convergence zone (ITCZ). Centers of high and low pressure have also been labeled. (Source of Original Modified Image: Climate Lab Section of the Environmental Change Research Group, Department of Geography, University of Oregon - Global Climate Animations).
The subtropical high pressure zone does not form a uniform area of high pressure stretching around the world in reality. Instead, the system consists of several localized anticyclonic cells of high pressure. These systems are located roughly at about 20 to 30° of latitude and are labeled with the letter H on Figures 7p-4 and 7p-5. The subtropical high pressure systems develop because of the presence of descending air currents from the Hadley cell. These systems intensify over the ocean during the summer or high Sun season. During this season, the air over the ocean bodies remains relatively cool because of the slower heating of water relative to land surfaces. Over land, intensification takes place in the winter months. At this time, land cools off quickly, relative to ocean, forming large cold continental air masses.
The sub polar lows form a continuous zone of low pressure in the Southern Hemisphere at a latitude of between 50 and 70° (Figures 7p-4 and7p-5). The intensity of the sub polar lows varies with season. This zone is most intense during Southern Hemisphere summer (Figure 7p-4). At this time, greater differences in temperature exist between air masses found either side of this zone. North of sub polar low belt, summer heating warms subtropical air masses. South of the zone, the ice covered surface of Antarctica reflects much of the incoming solar radiation back to space. As a consequence, air masses above Antarctica remain cold because very little heating of the ground surface takes place. The meeting of the warm subtropical and cold polar air masses at the sub polar low zone enhances frontal uplift and the formation of intense low pressure systems.
In the Northern Hemisphere, the sub polar lows do not form a continuous belt circling the globe (Figures 7p-4 and 7p-5). Instead, they exist as localized cyclonic centers of low pressure. In the Northern Hemisphere winter, these pressure centers are intense and located over the oceans just to the south of Greenland and the Aleutian Islands (Figure 7p-4). These areas of low pressure are responsible for spawning many mid-latitude cyclones. The development of the sub polar lows in summer only occurs weakly (Figure 7p-5 - over Greenland and Baffin Island, Canada), unlike the Southern Hemisphere. The reason for this phenomenon is that considerable heating of the Earth's surface occurs from 60 to 90° North. As a result, cold polar air masses generally do not form.
CITATION
Pidwirny, M. (2006). "Global Scale Circulation of the Atmosphere". Fundamentals of Physical Geography, 2nd Edition. 5/1/2012. http://www.physicalgeography.net/fundamentals/7p.html