STRUCTURE OF THE EARTH
The Earth is an oblate spheroid. It is composed of a number of different layers as determined by deep drilling and seismic evidence (Figure 10h-1). These layers are:
Figure 10h-1: Layers beneath the Earth's surface.
The core is a layer rich in iron and nickel that is composed of two layers: the inner and outer cores. The inner core is theorized to be solid with a density of about 13 grams per cubic centimeter and a radius of about 1220 kilometers. The outer core is liquid and has a density of about 11 grams per cubic centimeter. It surrounds the inner core and has an average thickness of about 2250 kilometers.
In this type of a collision, one of the plates is subducted under the other creating a deep oceanic trench. The Marianas trench in the Pacific ocean is created by the collision of the fast-moving Pacific Plate against the slower moving Philippine Plate. Convergence of two oceanic plates also creates chains of volcanic islands called island arcs. Island arcs are created by the friction of subduction which creates hot plumes of magma at the interface of the two plates. These hot plumes of magma then rise to the Earth's surface to form volcanoes. Another phenomena associated with collision and subduction of the plates is earthquakes. The gliding of one plate under the other is not smooth but jerky producing seismic waves.
The next diagram shows the collision of an oceanic and a continental plate (Figure 10i-6). In this illustration, the oceanic plate subducts under the lighter continental plate. Once again we get the formation of a trench, volcanoes, and earthquakes. Collision causes sediment deposited on the ocean floor to be piled up at the continental plate boundary. The creation of hot magma plumes also causes the continental crust to deform producing mountains.
Figure 10j-2: Formation of oceanic crust at rift zones located in the mid-oceanic ridges. Beneath the rift zone upwellings of magma occur in the mantle. These upwellings produce fissures and volcanoes on the ocean floor surface. The added rock, produced from the solidification of magma, pushes previously formed oceanic crust horizontally away from the rift zone like a conveyor belt. Ocean crust is returned to the mantle through subduction. This can occur when ocean crust meets continental crust or other ocean plates.
MOUNTAIN BUILDING
Figure 10k-2: The following illustration classifies the Earth's mountainous areas by elevation. The three elevation categories in this classification are: high (red), middle (orange), and low (yellow).
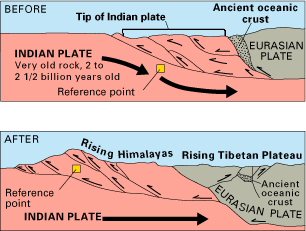
The Earth is an oblate spheroid. It is composed of a number of different layers as determined by deep drilling and seismic evidence (Figure 10h-1). These layers are:
- The core which is approximately 7000 kilometers in diameter (3500 kilometers in radius) and is located at the Earth's center.
- The mantle which surrounds the core and has a thickness of 2900 kilometers.
- The crust floats on top of the mantle. It is composed of basalt rich oceanic crust and granitic rich continental crust.
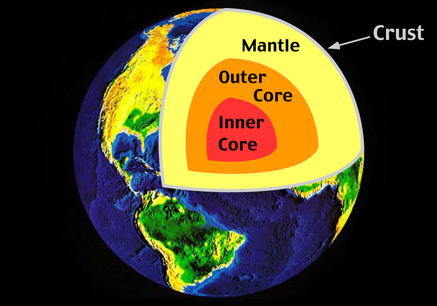
Figure 10h-1: Layers beneath the Earth's surface.
The core is a layer rich in iron and nickel that is composed of two layers: the inner and outer cores. The inner core is theorized to be solid with a density of about 13 grams per cubic centimeter and a radius of about 1220 kilometers. The outer core is liquid and has a density of about 11 grams per cubic centimeter. It surrounds the inner core and has an average thickness of about 2250 kilometers.
The mantle is almost 2900 kilometers thick and comprises about 83% of the Earth's volume. It is composed of several different layers. The upper mantle exists from the base of the crust downward to a depth of about 670 kilometers. This region of the Earth's interior is thought to be composed of peridotite, an ultramafic rock made up of the minerals olivine and pyroxene. The top layer of the upper mantle, 100 to 200 kilometers below surface, is called the asthenosphere.
Scientific studies suggest that this layer has physical properties that are different from the rest of the upper mantle. The rocks in this upper portion of the mantle are more rigid and brittle because of cooler temperatures and lower pressures. Below the upper mantle is the lower mantle that extends from 670 to 2900 kilometers below the Earth's surface. This layer is hot and plastic. The higher pressure in this layer causes the formation of minerals that are different from those of the upper mantle.
Scientific studies suggest that this layer has physical properties that are different from the rest of the upper mantle. The rocks in this upper portion of the mantle are more rigid and brittle because of cooler temperatures and lower pressures. Below the upper mantle is the lower mantle that extends from 670 to 2900 kilometers below the Earth's surface. This layer is hot and plastic. The higher pressure in this layer causes the formation of minerals that are different from those of the upper mantle.
The lithosphere is a layer that includes the crust and the upper most portion of the asthenosphere (Figure 10h-2). This layer is about 100 kilometers thick and has the ability to glide over the rest of the upper mantle. Because of increasing temperature and pressure, deeper portions of the lithosphere are capable of plastic flow over geologic time. The lithosphere is also the zone of earthquakes, mountain building, volcanoes, and continental drift.
The top most part of the lithosphere consists of crust. This material is cool, rigid, and brittle. Two types of crust can be identified: oceanic crust and continental crust (Figure 10h-2). Both of these types of crust are less dense than the rock found in the underlying upper mantle layer. Ocean crust is thin and measures between 5 to 10 kilometers thick. It is also composed of basalt and has a density of about 3.0 grams per cubic centimeter.
The continental crust is 20 to 70 kilometers thick and composed mainly of lighter granite (Figure 10h-2). The density of continental crust is about 2.7 grams per cubic centimeter. It is thinnest in areas like the Rift Valleys of East Africa and in an area known as the Basin and Range Province in the western United States (centered in Nevada this area is about 1500 kilometers wide and runs about 4000 kilometers North/South). Continental crust is thickest beneath mountain ranges and extends into the mantle. Both of these crust types are composed of numerous tectonic plates that float on top of the mantle. Convection currents within the mantle cause these plates to move slowly across the asthenosphere.
![]() |
Figure 10h-2: Structure of the Earth's crust and top most layer of the upper mantle. The lithosphere consists of the oceanic crust, continental crust, and uppermost mantle. Beneath the lithosphere is the asthenosphere. This layer, which is also part of the upper mantle, extends to a depth of about 200 kilometers. Sedimentarydeposits are commonly found at the boundaries between the continental and oceanic crust.
Isostacy
One interesting property of the continental and oceanic crust is that these tectonic plates have the ability to rise and sink. This phenomenon, known as isostacy, occurs because the crust floats on top of the mantle like ice cubes in water. When the Earth's crust gains weight due to mountain building or glaciation, it deforms and sinks deeper into the mantle (Figure 10h-3). If the weight is removed, the crust becomes more buoyant and floats higher in the mantle.
This process explains recent changes in the height of sea-level in coastal areas of eastern and northern Canada and Scandinavia. Some locations in these regions of the world have seen sea-level fall by as much as one meter over the last one hundred years. This fall is caused by isostatic rebound. Both of these areas where covered by massive glacial ice sheets about 10,000 years ago. The weight of the ice sheets pushed the crust deeper into the mantle. Now that the ice is gone, these areas are slowly increasing in height to some new equilibrium level.
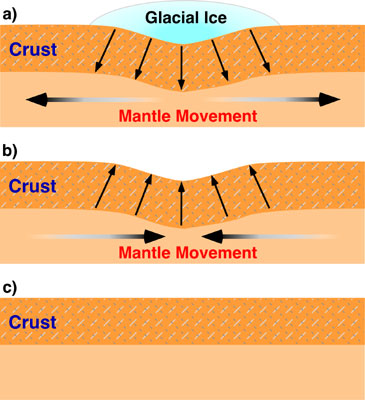
Figure 10h-3: The addition of glacial ice on the Earth's surface causes the crust to deform and sink (a).When the ice melts, isostatic rebound occurs and the crust rises to its former position before glaciation (b and c). A similar process occurs with mountain building and mountain erosion.
PLATE TECTONICS
PLATE TECTONICS
In the 19th and early 20th centuries, several scientists suggested that the continental masses had the ability to move across the Earth's surface. These early theories of continental drift were based on the following evidence:
- Locations of fossil occurrences suggested that some of the continental masses may have been connected in the geological past.
- Paleo climatic evidence indicates that now tropical regions on some continents had polar climates in the past. This may indicate that these regions were located at different latitudes.
- Some continents seem to fit together like a jigsaw puzzle.
- Some geologic deposits of rocks on the East coast of North and South America are similar to deposits found on the West coast of Africa and Europe.
During the first 30 years of this century the theory of continental drift was actively debated among geo-scientists. However, during the following 30 year period, debate on this theory waned because of the inability of scientists to propose a mechanism to cause the movement of the continental masses.
In the 1960s, the theory was resurrected with the discovery of alternating patterns of rock magnetism in surface sea-floor rocks. Scientists had previously discovered that the magnetic orientation of certain crystals in rocks varies from normal to reversed polarity depending on the date that the rock was formed and solidified. It was also discovered that these magnetic reversals were common and occurred on a regular basis. The polarity patterns found in the rocks at the ocean floor seemed to mirror themselves either side of the mid-oceanic ridge found at the centers of the ocean basins. Further, geologic dating of the rocks indicated the age of the sea-floor rocks increase as one moved away from the mid-oceanic ridge (see Figure 10i-1).
Based on this information, scientists developed the theory of sea-floor spreading which suggested that volcanic rift zones at the mid-oceanic ridge represent areas of crustal creation. The following diagram illustrates the process of crustal creation and the magnetic striping process. In Figure 10i-2, illustrations "a" to "c" represent a sequence in time from the past to the present. In illustration a, rocks of normal polarity are being deposited at the rift zone located along the mid-oceanic ridge.
As new rock is created, older rock is pushed away from the ridge. The reversed polarity rock shown in this diagram was created before the current normal polarity layer. Illustration b shows the process some time later. In this diagram, we now have four layers of rock with alternating polarity. By the third illustration, sea-floor spreading and changes in magnetic polarity have created six recognizable layers of rock either side of the rift zone.
As new rock is created, older rock is pushed away from the ridge. The reversed polarity rock shown in this diagram was created before the current normal polarity layer. Illustration b shows the process some time later. In this diagram, we now have four layers of rock with alternating polarity. By the third illustration, sea-floor spreading and changes in magnetic polarity have created six recognizable layers of rock either side of the rift zone.
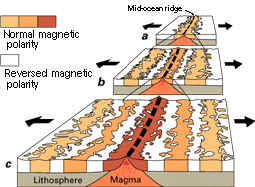
Figure 10i-2: Creation of oceanic crust on the ocean floor. (Source: U.S. Geological Survey). |
The theory of sea-floor spreading started a revolution in the Earth Sciences. Subsequent research discovered that the Earth's surface was composed of a number of oceanic and continental plates that float on top of the asthenosphere (see Figure 10i-3). Other research suggested that convection currents within the Earth's mantle were responsible for the creation of oceanic crust and the drifting of the continents (Figure 10i-4). In this diagram, it is theorized that convection currents within the Earth's mantle cause the creation of new oceanic crust at the mid-oceanic ridges. Oceanic crust is destroyed at areas where this crust type becomes subducted under lighter continental crust. This process also creates the deep oceanic trenches.
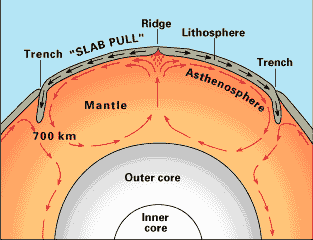
Figure 10i-4: Convection currents in the Earth's mantle and their role in oceanic crust formation and destruction. (Source: U.S. Geological Survey).
|
The theory of plate tectonics offered new and more scientifically sound explanations for a number of observed geologic phenomena. For example, the following diagrams illustrate the three types of plate convergence and describe some of the geologic repercussions of these processes. The first diagram models the tectonic convergence of two oceanic plates (Figure 10i-5).
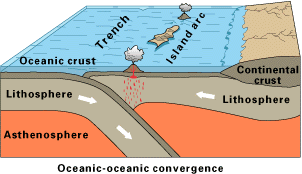
Figure 10i-5: Collision of two oceanic plates. (Source: U.S. Geological Survey). |
In this type of a collision, one of the plates is subducted under the other creating a deep oceanic trench. The Marianas trench in the Pacific ocean is created by the collision of the fast-moving Pacific Plate against the slower moving Philippine Plate. Convergence of two oceanic plates also creates chains of volcanic islands called island arcs. Island arcs are created by the friction of subduction which creates hot plumes of magma at the interface of the two plates. These hot plumes of magma then rise to the Earth's surface to form volcanoes. Another phenomena associated with collision and subduction of the plates is earthquakes. The gliding of one plate under the other is not smooth but jerky producing seismic waves.
The next diagram shows the collision of an oceanic and a continental plate (Figure 10i-6). In this illustration, the oceanic plate subducts under the lighter continental plate. Once again we get the formation of a trench, volcanoes, and earthquakes. Collision causes sediment deposited on the ocean floor to be piled up at the continental plate boundary. The creation of hot magma plumes also causes the continental crust to deform producing mountains.
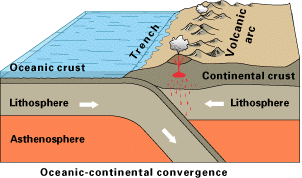
Figure 10i-6: Collision of a oceanic plate with a continental plate. (Source: U.S. Geological Survey). |
In the final illustration two continental plates collide (Figure 10i-7). Once again one of the crustal plates is subducted under the other producingearthquakes. A mountain range is produced at the plate boundaries because of the deformation of rocks. Some of the rocks in the mountain range may be sedimentary and may have been set down in an ocean environment that existed between the two continental crusts prior to collision.
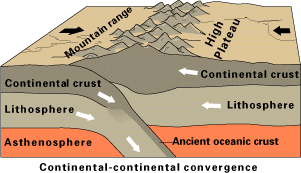
Figure 10i-7: Collision of two continental plates. (Source: U.S. Geological Survey). |
In summary, modern plate tectonic theory states that the surface crust of the Earth is composed of many independent segments called plates. These plates have the ability to move horizontally by gliding over the plastic asthenosphere. In some cases, plates can collide with each other at the plate boundaries causing subduction and the production of earthquakes, volcanoes, mountain building, and oceanic trenches. At other plate boundaries, plates may move away from each other because of sea-floor spreading or horizontally move past one another creating transform faults and earthquakes.
CRUSTAL FORMATION PROCESSES
Studies of seismic waves have discovered that the Earth's crust consists of two basic types. Beneath the oceans we find a crust that is on average 7 kilometers thick and composed mainly of basalt. Oceanic crust also has a density of about 3.0 grams per cubic centimeter. The continent's are composed of mainly granitic rock whose thickness varies between 10 and 70 kilometers. The thickest portions of continental crust are found under the various mountain ranges.
Continental crust is also lighter than oceanic crust having a density of about 2.7 grams per cubic centimeter. Oceanic and continental rocks also differ from each other in terms of age. Continental crust contains some very old rocks that were formed during the Precambrian between 3 and 4 billion years ago. Oceanic rocks are normally quite young deposits. Isotopic dating of the rocks found on the sea-floor indicates that they were created less that 180 million years ago.
Continental crust is also lighter than oceanic crust having a density of about 2.7 grams per cubic centimeter. Oceanic and continental rocks also differ from each other in terms of age. Continental crust contains some very old rocks that were formed during the Precambrian between 3 and 4 billion years ago. Oceanic rocks are normally quite young deposits. Isotopic dating of the rocks found on the sea-floor indicates that they were created less that 180 million years ago.
Variations in the age, density, and chemical composition of oceanic and continental crust suggest that these lithospheric deposits were created by different processes. The following discussion describes these differences.
Continental Crust
All of the Earth's continents have a core foundation that is made of mixtures of very old granite, gneiss, schist, sedimentary, and volcanic rocks. This core foundation is often referred to as a shield or basement rock. Rocks found in the shields were formed during the Precambrian and are some of the oldest rocks found on the Earth. In Canada, some of the metamorphic rocks have been dated to an age of 3.96 billion years. Geologists believe that the major continental cores were formed by the early solidification of the lighter components of magma between 3.9 and 3.8 billion years ago. The continental shields are generally covered by younger sedimentary deposits.
These sedimentary rocks constitute the interior platforms of the continents. The oldest platform rocks were laid down in shallow seas about 600 million years ago. In central North America, the platform sedimentary deposits are between 1000 to 2000 meters thick. Together the shield and platform form what geologists call a craton. Most of the Earth's continental cratons have been tectonically stable and have experienced little deformation for hundreds of millions of years.
Around the edge of the continental cratons are the continental margins. The continental margins are primarily composed of sedimentary rocks. These sedimentary rocks were originally laid down in the oceans. Tectonic collisions and plate subduction caused the accretion of these deposits at the edges of the continental cratons. In some cases, this accretion is modified by the processes of tectonic compression,folding, and faulting to produce mountain ranges.
Igneous Activity and the Continents
Materials are also added to continental crust through intrusive and extrusive igneous activity. Plumes of magma from the Earth's asthenosphere are generated from the friction produced at the contact zone where oceanic crust slides past continental crust (Figure 10j-1). These plumes then rise upward into continental crust to form granitic plutons or a variety of volcanic features on the Earth's surface. A pluton can be defined as any igneous intrusion of rock that forms a kilometer or more below the Earth's surface. The diagram (Figure 10j-2) below illustrates some of the features associated with igneous intrusions or plutons. Some of the major features include:
- Dyke: thin vertical veins of igneous rock that form in the fractures found within the crust. Because these intrusive features cool quickly their rocks are dominated with fine mineral grains.
- Sill: horizontal planes of solidified magma that run parallel to the grain of the original rock deposit.
- Batholith: large plutonic masses of intrusive rock with more than 100 square kilometers of surface area.
- Volcanic Pipe: if a dyke reaches the surface of the Earth it is then called a volcanic pipe. Igneous deposits produced by this feature are extrusive in nature.
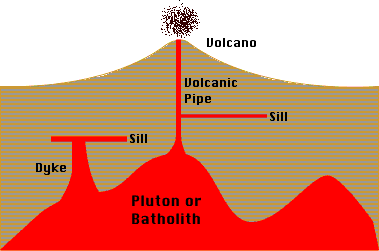
Figure 10j-2: Common plutonic features.
Plumes that are able to reach the Earth's surface produce volcanoes. Most of the continental volcanoes found on our planet are located along the edge of the continents where oceanic crust is being actively subducted. In North America, the zone of active volcanoes is located on the west coast where the subduction of the Pacific plate occurs. Volcanoes add mass to the continents when magma produces lava flows, tephra, and volcanic ash.
Oceanic Crust
Unlike continental crust, oceanic crust is actively being created at the various mid-oceanic ridges. At the mid-ocean ridges, magma erupts onto the ocean floor in centrally located rift zones (Figure 10j-2). The newly added rock then horizontally pushes previously created ocean crust away from the rift in a conveyor belt fashion. Because of this process, we find that the age of oceanic crust increases as we move away from the rift zone. When the oceanic crust encounters a slab of continental crust it becomes subducted because of its greater density. This process causes the oceanic crust to return to the mantle were it is re-melted into magma. The process also causes the movement of continental crust across the surface of the Earth.
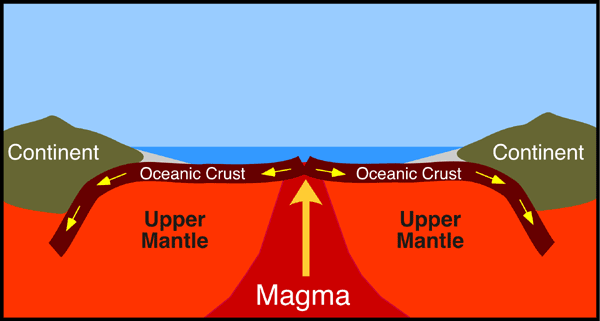
Figure 10j-2: Formation of oceanic crust at rift zones located in the mid-oceanic ridges. Beneath the rift zone upwellings of magma occur in the mantle. These upwellings produce fissures and volcanoes on the ocean floor surface. The added rock, produced from the solidification of magma, pushes previously formed oceanic crust horizontally away from the rift zone like a conveyor belt. Ocean crust is returned to the mantle through subduction. This can occur when ocean crust meets continental crust or other ocean plates.
MOUNTAIN BUILDING
A mountain can be defined as an area of land that rises abruptly from the surrounding region. A mountain range is a succession of many closely spaced mountains covering a particular region of the Earth. Mountain belts consist of several mountain ranges that run roughly parallel to each other. The North American Cordillera, the Himalayas, the Alps, and the Appalachians are all examples of mountain belts that are composed of numerous mountain ranges.
Some mountains are volcanic in origin forming where rising magma breaks through the Earth's surface. Volcanic mountains tend to have sporadic distributions within a mountain range (Mount St. Helens, Rainier, and Baker) or can occur alone because of a localized hot spot(Hawaiian Islands). Most mountains were created from tectonic forces that elevate, fold, and fault rock materials. Tectonic mountains can occur as a single range (the Urals) or as a belt of several mountain ranges (North American Cordillera). Figures 10k-1 and 10k-2 show the location of some of the major mountain systems found on the Earth's surface. These major mountain systems include the North American Cordillera, Andes, Alps, Urals, Appalachians, Himalaya, Caledonian Belt, and the Tasman Belt.
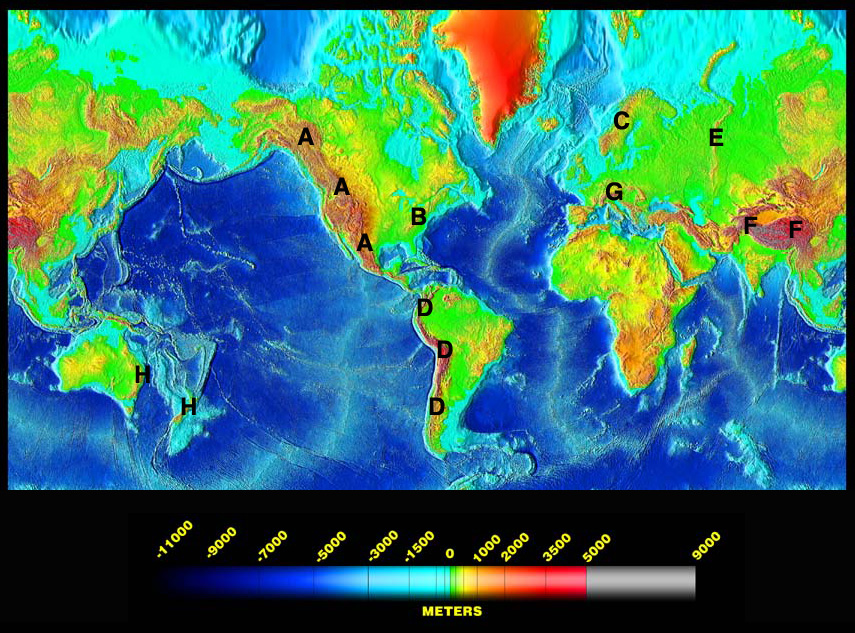
Figure 10k-1: This image shows the topography of both land and ocean surfaces. Elevation is indicated by color. The legend below shows the relationship between color and elevation. The Earth's major mountain systems are generally colored orange to red to grey. Some of the major mountain belts on the Earth are the North American Cordillera (A), Appalachians (B), Caledonian Belt (C), Andes (D), Urals (E), Himalaya (F), Alps (G), and the Tasman Belt (H). (Source of Modified Image: NOAA, National Geophysical Data Center).
The Earth's mountain ranges have various ages of formation. Parts of the Himalayas are relatively quite young. Mountain building in this region of the world began about 45 million years ago when the continental plates of India and Eurasia converged on each other. The Himalaya mountains are still actively being uplifted. The Appalachian belt is quite old. Mountain building in this region of the world started about 450 million years ago. Orogeny stopped in the Appalachians about 250 million years ago.
The long passage of time without active uplift has allowed weathering and erosion to remove large amounts of bedrock from the Appalachians. These processes have also significantly lowered and rounded the peaks of the various mountains found in this belt. Mountain building episodes in the North American Cordillera have been occurring over a very long period of time and still continue today. Some sedimentary rocks in the Rocky Mountain range (located on the eastern edge of the North American Cordillera) date to over a billion years old.
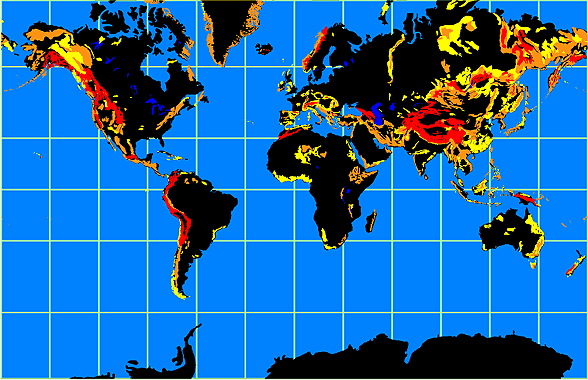
Figure 10k-2: The following illustration classifies the Earth's mountainous areas by elevation. The three elevation categories in this classification are: high (red), middle (orange), and low (yellow).
Evolution of Mountains
Geologists have developed a general model to explain how most mountain ranges form. This model suggests that mountain building involves three stages: (1) accumulation of sediments, (2) an orogenic period of rock deformation and crustal uplift, and (3) a period of crustal uplift caused by isostatic rebound and block-faulting. The later two stages of this model involve tectonic convergence of crustal plates which provides the compressional and tensional stresses that produce rock deformation, uplift, and faulting.
Mountain belts normally contain numerous layers of sedimentary and volcanic igneous rocks. These accumulations can be several kilometers in thickness. Most of these accumulations were originally deposited in a marine environment. The beds of the sedimentary rocks are composed of particles that came from nearby terrestrial landmasses. These particles were released from rocks by weathering and then transported by erosional forces to the edge of the terrestrial continental crust. Beyond the edge of the continents, these sediments are lithified to form shales, limestones, and sandstones that make up the continental shelves, slopes, and rise. Accumulations of volcanic rock develop along convergent boundaries where subduction is causing magma plumes to form plutons and volcanoes. The volcanoes are usually spatially organized in a line, called an island arc, that runs at right angles to the direction of crustal movement.
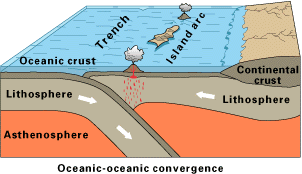
Figure 10k-3: Volcanic rocks found in mountains often originate from magma plumes that have migrated up through oceanic crust. The subduction of one oceanic plate under another creates friction that melts rock into magma. This magma then migrates upward through the crust forming plutons and volcanoes. Volcanic rocks can also be converted into sedimentary sandstones. Weathering and erosion can remove material from terrestrial volcanic deposits to marine depositional environments. Overtime these sediments can then become lithified. (Source: U.S. Geological Survey). |
In the orogenic stage of mountain building, the accumulated sediments become deformed by compressional forces from the collision of tectonic plates. This tectonic convergence can be of three types: arc-continent, ocean-continent or continent-continent. In an ocean-continentconvergence, the collision of ocean and continental plates causes the accretion of marine sedimentary deposits to the edge of the continent. Arc-continent convergence occurs when an island arc collides with the edge of a continental plate.
In this convergence, the ocean plate area between the arc and the continent is subducted into the asthenosphere and the volcanic rocks and sediments associated with the island arc become accreted to the margin of the continent over time. This type of collision may have been responsible for the creation of the Sierra Nevada mountains in California during the Mesozoic Era. The final type of convergence occurs when an ocean basin closes and two continental plates collide. Continent-continent convergence mountain building is responsible for the formation of the Himalayas, Ural, and Appalachian mountain systems.
In this convergence, the ocean plate area between the arc and the continent is subducted into the asthenosphere and the volcanic rocks and sediments associated with the island arc become accreted to the margin of the continent over time. This type of collision may have been responsible for the creation of the Sierra Nevada mountains in California during the Mesozoic Era. The final type of convergence occurs when an ocean basin closes and two continental plates collide. Continent-continent convergence mountain building is responsible for the formation of the Himalayas, Ural, and Appalachian mountain systems.
In all three types of tectonic convergence, layered rocks that were once located in the ocean basin are squeezed into a smaller and smaller area. This compression causes the once flat sedimentary beds to be folded and uplifted. When the compressional forces become greater than the rocks ability to deform, faulting occurs. Compressional forces typically result in reverse and overthrust faulting. Another consequence of the orogenic stage is regional metamorphism and the incursion of magma plumes, plutons, and volcanoes into the growing mountain range.
Figure 10k-4 illustrates how the collision Eurasian and Indian plates created the Himalayas. In this orogeny, compressional forces squished sedimentary deposits that existed between the converging continental plates and rocks at the margin of the Eurasian and Indian plates upward in elevation. These forces also created a number of overthrust faults.
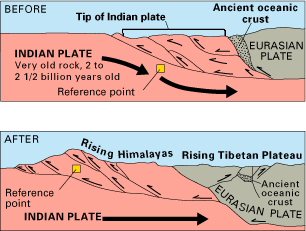
Figure 10k-4: Formation of the Himalaya Mountains. Compressional forces due to the collision of the Eurasian and Indian continental plates caused ocean sediments and continental rocks to be pushed upward in elevation. (Source: U.S. Geological Survey).
At the end of plate convergence, mountain building enters its final stage. This stage is characterized by crustal uplift because of isostatic rebound and block-faulting (Figure 10k-5). Isostatic rebound involves the vertical movement of continental crust that is floating in the plastic upper mantle. As erosion removes surface materials from mountains, the weight of the crust in this region becomes progressively less. With less weight, the continental crust makes an isostatic adjustment causing it to rise vertically (float higher) in the mantle. This process also causes tensional forces to exist in a horizontal direction breaking the continental crust into a number of blocks. Each block moves vertically to compensate for the tensional forces producing normal and graben faults.
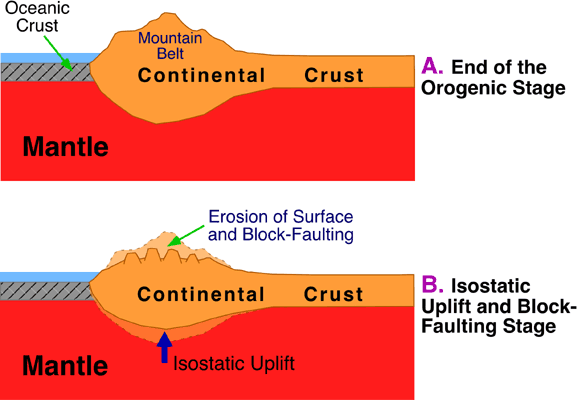
Figure 10k-5: After the orogenic stage, weathering and erosion begin removing material from the surface of the newly created mountains. The removal of rock mass makes the area of the continental crust where the mountains are less heavy and that end of the crust begins to float higher in the mantle. This isostatic rebound causes vertical uplift and the tensional forces due to the movement of the crust creates normal and graben faults. |
CITATION
Pidwirny, M. (2006). Fundamentals of Physical Geography, 2nd Edition. 01/01/2012. http://www.physicalgeography.net/fundamentals/10h.html